DOI: 10.7256/2453-8922.2023.1.40136
EDN: RDPROK
Received:
05-04-2023
Published:
22-04-2023
Abstract:
The subject of the study was the soil and geochemical features of southern tundra cryogenic landscapes on the Khanovei educational and scientific field site located nearby the eponymous settlement in the Vorkuta district, Komi Republic. Morphology of soil profiles were described according to the modern Russian soil classification system. Chemical analytical sample treatment was done to evaluate such physical and chemical parameters of soil horizons as the pH rate, water-soluble salts content, organic carbon content, soil texture, iron fractional composition. The main point among soil and geochemical features of the Khanovei field site was gross chemical composition of cryogenic soils. Gross concentrations of chemicals were measured with the X-ray fluorescence method by portable X-ray Fluorescence Analyzer and then geochemical ratios of radial (profile) and lateral (catenar) differentiation of chemical elements – R and L. Largest R ratio meanings in soil profiles were in gleyic horizons including above-permafrost. Peat horizons without traces of soil mineral fraction were depleted with the most of elements (R = 0,1–0,3) and mineral horizons of soils were differed by increased RFe, RMn etc. The lower part of the catena with soils of mostly sandy texture was depleted by the main part of elements (Ti, Zn, Zr etc.) and predominantly loamy-textured soils in the upper part accumulate these elements and form increased L ratio meanings.
Keywords:
cryogenic soils, soil-geochemical catena, macro- and microelements, lateral differentiation, radial differentiation, Bolshezemelskaya tundra, tundra soils, Vorkuta area, Khanovei scientific and educational field site, Republic of Komi
This article is automatically translated.
You can find original text of the article here.
1. IntroductionIn the north of the European territory of Russia, permafrost rocks (MMP) are mainly distributed intermittently in the cryolithozone[7], as a result of which a large number of different geocryological settings are created in various landscape conditions [22,25], and when moving along the Bolshezemelskaya tundra from south to north, landscape diversity increases[14]. The Khanovey educational and scientific training ground, located in the landscape subzone of the southern tundra, is characterized by a high variety of permafrost-landscape environments spread over a small area, as a result of which studies of the patterns of the spread of MMP and their accompanying natural processes, including soil-geochemical ones [3,5,19], become possible here without significant labor costs. The melting of MMP as a result of climatic changes is especially pronounced in the area of the southern border of the cryolithozone[4]. An increase in the capacity of the seasonally thawed layer (STS) of soils from year to year contributes to an increase in the depth of the active migration of chemicals and a decrease in the contrast of profile distributions of the content of chemical elements. 2. Objects and methods2.1. Physical and geographical conditions of the research area Geographical location The research area is located on the gently sloping over-floodplain terrace of the Vorkuta River (south-east of the Bolshezemelskaya tundra) in its middle course (67°17’9.05” s.w., 63°39’25.38” v.d., altitude above sea level = 150 m). UNP Hanovey is a section of a gentle slope with an area of about 0.5 km 2, bounded on the west by the Moscow–Vorkuta railway line, on the east by the Vorkuta River cut. The nearest settlement to the landfill is the village of Hanovey with the railway station of the same name adjacent to the landfill from the southwest. The research area is 30 km to the southwest from Vorkuta (Fig. 1). 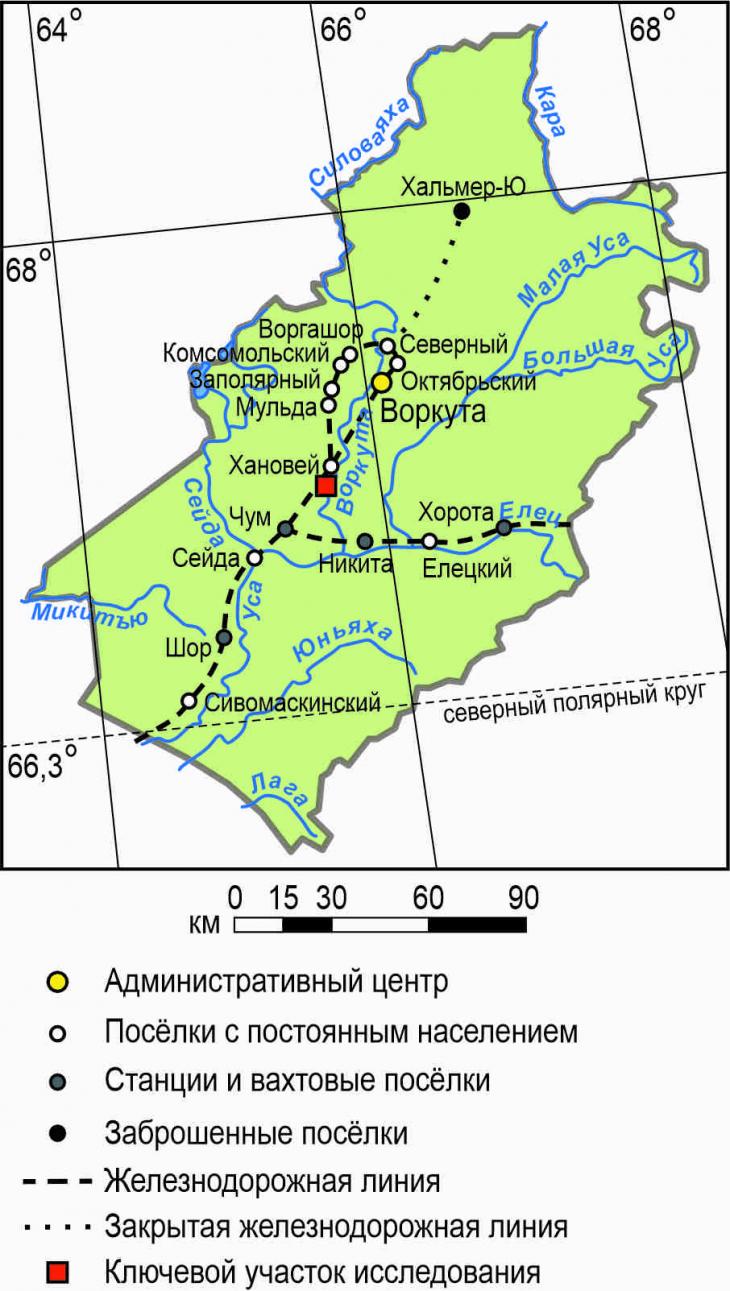 Fig. 1. Location of UNP Hanowei Physical and geographical characteristics of the research areaThe largest area on the territory of Komi is occupied by the vast Pechora lowland of the valleys of the Pechora and Usy rivers [13]. The most ancient deposits are Early Precambrian (more than 2 billion years old), and the surface is composed of glacial and postglacial boulder loams and sands with alluvial and lake-marsh sands, sandy loams and loams [1,8]. The macroforms of the relief were formed as a result of the manifestations of irregularities in the roof of the bedrock deposits, and the mesorelief was formed under the active action of exogenous processes – erosion and accumulation. These include ridge-type hills (musyura), wide, well-developed river valleys, as well as combinations of convex peat mounds of heaving (palza) with overgrown thermokarst depressions.[9] Permafrost rocks (MMP) have a discontinuous (50-95% of the area) and massively insular (10-50%) distribution here (Fig. 2). The temperatures of the MMP of the discontinuous distribution are characterized by a range of average temperatures from 0.5 to -2.0 ° C, massively insular – from +1 to -1 ° C. The thickness of the seasonal melt layer (STS) here reaches 1-1.7 m, depending on the granulometric composition of the substrate.[25] The nature of the vegetation cover has a significant impact on the depth of freezing and thawing. 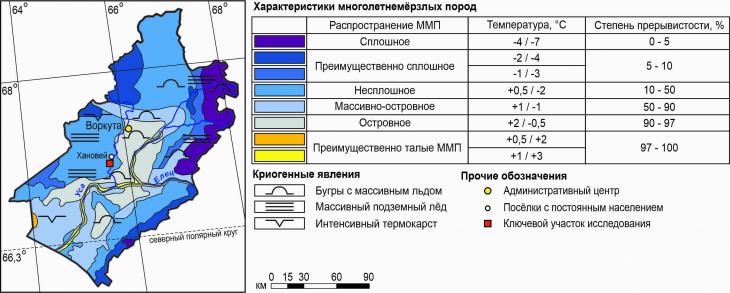 Fig. 2. Geocryological map of the Vorkuta district of the Komi Republic, according to [25] The climate of the Vorkuta district belongs to the subarctic type. The average annual air temperature is -5.4 °C, in July it warms up to +13.2 °C, and the coldest month is January with a temperature of -19.5 °C (according to the Vorkuta meteorological station [12]). The duration of the growing season here is 92 days, and the sum of active temperatures reaches 493 ° C. The humidity of the air is almost the same throughout the year and is 81%. On average, 507 mm of precipitation falls per year. The thickness of the snow cover here varies from 53 to 180 cm . Interstitial spaces are occupied by soils of the gleezem type, and cryometamorphic soils are developed on gentle slopes [3, 19]. The different thickness of the peat horizon at the landfill provides classification differences between cryozems (O – CR – C(g) profile) and peat-cryozems (T – CR – C(g) profile), gleezems (O – G – CG profile) and peat-gleezems (T – G – CG) [11]. On soils of lighter granulometric composition, shrubby-green moss lichen tundras are found, on loamy soils, grass-shrubby-sphagnum tundras are developed in complexes with pushchitsa-sedge-sphagnum swamps.[6,18] The physical and geographical conditions were described in more detail in our work.[5] 2.2. Field research methodsField work was carried out on UNP Hanowei and adjacent territories in September 2022. In the course of the research, 10 soil sections were laid in various landscape conditions from the low floodplain of the Vorkuta River to the slightly convex surface of the interfluve plain (Fig. 2).
9 of the 10 sections laid down were the soil-geochemical catena of the right bank of Vorkuta. Soil sections were laid using shovels. In case of reaching the upper limit of the MMP, samples of frozen rocks were knocked out of the thickness with an axe. Samples of seasonally thawed soil horizons were collected using knives in zip-lock plastic bags. The total mass of each sample was 250-500 g. In the field, soil horizons were described according to the scheme proposed for a comprehensive description in the Classification and Diagnostics of soils in Russia[11] and the Field Soil Determinant.[17] Field names of soils were also given based on combinations of diagnostic genetic horizons and features specified in the Field Soil Determinant.[17] 2.3. Laboratory research methodsIn the packed state, the samples were delivered to the chemical and analytical laboratory of the Ecological and Geochemical Center (EGC) of the Geographical Faculty of Moscow State University (Moscow). They were dried at room temperature to an air-dry state, after which they were subjected to appropriate sample preparation procedures for further laboratory study of the chemical composition and physico-chemical properties. The following analytical indicators of soils were analyzed in laboratory conditions: acidity of aqueous extract (pH), total content of easily soluble salts (TDS), total organic carbon content (C org.), granulometric composition of soils (indicating the class in accordance with N.A. Kachinsky's systematics), concentrations of non-silicate (Fe hc) and oxalate-soluble (Fe a) iron in soils, as well as the gross content of macro- and microelements. Acidity of water extractsTo analyze the acidity of soils, the sample was ground with a porcelain pestle in a porcelain mortar, and then sifted through a sieve with a pore diameter of 1 mm. The acidity was measured with an ion-selective METTLER TOLEDO electrode in a suspension made in a ratio of soil to distilled water of 1:2.5, and in the case of analysis of organogenic horizons, the ratio decreased to 1:25. Total content of easily soluble salts Sample preparation for the analysis of the content of easily soluble salts was similar to that described above for the study of soil acidity. The content of water-soluble salts in soils was studied by a METTLER TOLEDO laboratory conductometer in a suspension made in a ratio of soil to distilled water of 1:2.5. Total organic carbon content The concentrations of organic carbon in soils were investigated by I.V. Tyurin's method with photometric termination. To do this, the soil sample was ground with a porcelain pestle in a porcelain mortar, then sifted through a sieve with a pore diameter of 0.25 mm. A soil sample weighing 1 g weighed on analytical laboratory scales was filled with 10 ml of 0.4 M potassium bichromate (K 2 Cr 2 O 7) and placed in a drying cabinet heated to +150 ° C for 40 minutes. Then the extract was cooled at room temperature and titrated with 0.2 M solution of More salt (double sulfuric acid salt of iron and ammonium - FeSO ?·(NH ?)? SO ?·6H ? O) in the presence of 5-6 drops of phenylanthranilic acid (C 13 H 11 NO 2). The end point for this type of redox titration is the change of color of the solution from reddish-brown to dark green. The calculation of the organic carbon content was carried out according to the formula:  where V 1 is the volume of the Mohr salt solution used for titration of the blank sample (samples without a sample) of the soil (cm 3); V 2 is the volume of the Mohr salt solution used for titration of the sample with a soil sample (cm 3); M is the molarity of the Mohr salt solution; 0.003 is the molar mass of ? S (g/mol); 100 is the conversion factor per 100 g of soil; m is the mass of the dry soil sample. Granulometric composition The soil sample for granulometric analysis was ground with a rubber pestle in a porcelain mortar and sifted through a sieve with a pore diameter of 1 mm. The granulometric composition of soils was studied by laser granulometry after grinding the sample in a porcelain cup with a rubber pestle with a solution of sodium pyrophosphate (Na 4 P 2 O 7). Pyrophosphate is used to eliminate the adhesion of soil particles. The analysis of the granulometric composition of soils was measured using a laser granulometer Fritsch Analysette-22 (Germany). To classify soils according to the content of the fraction of physical clay in them, the N.A. Kaczynski scale was used (Table 1). Table 1. Classification of soils of podzolic type of soil formation according to the content of the fraction of physical clay (N.A. Kachinsky) Granulometric composition classPhysical clay content, % | Sandy | Loose-sandy | |
0 – 5 | Svyazno-sandy | 5 – 10 | Sandy loam | 10 – 20 | LoamyLight loamy | | 20 – 30 | Medium Loamy | 30 – 40 | Heavy loamy | 40 – 50 | ClayLight - clay | | 50 – 65 | Medium clay | 65 – 80 | Heavy clay | >80 | Concentrations of non-silicate and oxalate-soluble iron
Determination of dithionite-soluble iron compounds was performed by the Mera-Jackson method. A sample of air-dry soil sifted through a sieve with a diameter of 1 mm holes, weighing 2 g, was placed in a centrifuge tube with a capacity of 50 cm3. 20 ml of 0.3M citrate sodium solution (Na 3 C 6 H 5 O 7) and 2.5 ml of 1M sodium bicarbonate solution (NaHCO 3) were poured into the test tube, stirred in a circular motion and heated in a water bath to 800 ° C. Then 0.5 g of dry powder of sodium dithionite (Na 2 S 2 O 4) was introduced into the test tube and mixed with a glass rod. After that, 5 ml of saturated sodium chloride (NaCl) solution was added to the test tube, mixed and left in a water bath until a flake-like precipitate was formed. Then centrifugation was carried out for 10 minutes at 3000 rpm, the centrifuge was drained through a dry dense filter into a 250 ml volumetric flask. Soil treatment in a centrifuge tube was repeated two more times, collecting centrifugates in the same measuring flask. After cooling, the contents of the measuring flask were brought to the mark with distilled water and mixed. The concentration of iron in the resulting solution was determined by atomic absorption spectroscopy. To analyze the group composition of iron, the soil sample was ground with a porcelain pestle in a porcelain mortar, and then sifted through a sieve with a pore diameter of 1 mm. The extraction of crystallized iron compounds from soils was carried out by the method of F. Tamma, based on the extraction of crystallized iron using a Tamma buffer solution (0.14 M by H 2 C 2 O 4 and 0.2 M by (NH 4)2 C 2 O 4). A soil sample filled with a buffer solution weighing 1 g was filtered through a folded paper filter "blue ribbon" with a pore diameter of 0.45 microns. The filtration procedure was repeated 3 times, after which the solution was diluted with distilled water in a 250 ml volumetric flask and poured into plastic tubes. The concentrations of oxalate-soluble forms of iron were analyzed by atomic absorption spectroscopy. Gross contents of macro- and microelements Concentrations of macro- and microelements were measured in soil samples ground in an agate mortar and sifted through a sieve with a pore diameter of 0.25 mm by X-ray fluorescence analysis (XFA). For this purpose, a portable mining and geological X-ray fluorescence analyzer Olympus Delta Professional (USA) was used. This device is capable of determining the gross concentrations of 35 chemical elements with atomic masses from Mg to U. 2.4. Geochemical landscapes and coefficientsDescriptions and characteristics of geochemical features of the landscape are given in accordance with the works of A.I. Perelman and N.S. Kasimov[16]. To characterize elementary geochemical landscapes, they were given names in accordance with the classification of M.D. Bogdanova and co-authors[2]. The forms (and elements) of the relief are used here as the main criterion for the allocation of elementary landscapes. Geochemical coefficients of radial and lateral differentiation were used to characterize the spatial heterogeneity of the chemical composition of soils.[15,16] The radial differentiation coefficient (R) is used to study the profile (vertical) heterogeneity of the chemical composition by comparing the concentrations of specific substances in soil horizons with those in the horizon of the soil-forming rock:  where R x(mountains) is the concentration of chemical substance x in the soil horizon; R x (pores) is the concentration of chemical substance x in the soil-forming rock. The lateral differentiation coefficient (L) is used to study the catenary (subhorizontal) heterogeneity of the chemical composition of the landscape. In this case, a comparison is made between the concentration of chemical substance x in the soils of subordinate and autonomous (eluvial) elementary geochemical landscapes. This indicator is intended to characterize the lateral migration of chemicals in soils connected by single streams of moisture moving along the relief from top to bottom under the influence of gravity. The soil-geochemical catena acts as a model area when using L:  where Lx(sub) is the concentration of chemical substance x in a subordinate elementary geochemical landscape; L x (auth.) is the concentration of chemical substance x in an autonomous (eluvial) elementary geochemical landscape. When analyzing the catenary heterogeneity of the chemical composition of soils in the Vilyuya Valley, data on the L coefficients of seasonally thawed and permafrost soil horizons are presented in parallel. 3. Results3.1. Soil-geochemical structure of the Khanovey educational and scientific landfillThe cryogenic soils found at the Khanoveysky UNP are represented by profiles of the types cryometamorphic, cryozems, peat-gleezems, peat, as well as gray humus and alluvial. Among the subtypes, gley and gley are widely distributed, since reducing environmental conditions often develop in the permafrost horizons of loamy granulometric composition, which leads to morphological manifestations of iron reduction. Cryogenically-hardened subtypes of soils, also often found here, indicate the manifestation of the closure of the lower boundary of the MMP with the freezing front from above, in the place of which the oxidized form of iron is concentrated.[11] The conditions of soil formation and the morphological structure of soil profiles are considered in detail in our work.[5]
The soil-geochemical catena laid on the left bank of the Vorkuta River represents elementary geochemical landscapes sequentially located from the watershed surface to the low floodplain of Vilyuya (Fig. 3): eluvial of the first order with a predominance of radial migration of matter (E 1R) landscape of the watershed surface, eluvial landscapes of the second order (E 2) above–floodplain terraces - the third and second, transeluvial landscapes with weak (TE1) and intense (TE3) outflow on the slopes of above-floodplain terraces, the surface of the first above-floodplain terrace, covered with a layer of eluvial material and poorly expressed in relief, is still isolated and represents a transeluvial-accumulative landscape (TEA), and below the relief is the surface of a low floodplain – a superaqual transit (ST3) landscape. The aquatic landscape (A) of the Vorkuta River is the final one in the catenary chain, however, consideration of its geochemical specificity is not included in the objectives of this study. 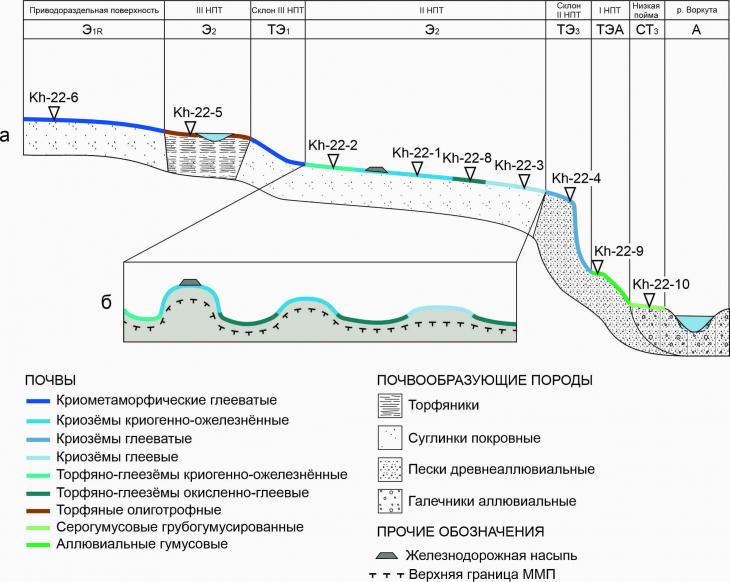 Fig. 3. Soil-geochemical catena at the Khanovey educational and scientific training ground (a) and microstructure of the soil cover of the first above-floodplain terrace (b) The upper parts of the soil-geochemical catena on relief elements with improved drainage, MMP depth of more than 1 m, as well as loamy granulometric composition of soil-forming rocks (Fig. 3a) are characterized by areas of cryometamorphic soils (the dividing surface, the slope of the third above-floodplain terrace). On the third and second above-floodplain terraces, deep-thawing (more than 2 m) peat deposits are developed, on the third their thickness exceeds 2 m (Fig. 3a), which makes it possible to allocate an area of peat oligotrophic soils on it. On the second above–floodplain terrace, the peat thickness does not exceed 0.5 m, and loamy soil-forming rocks lie below (the upper boundary of the MMP is located at a depth of about 0.6-0.7 m), which corresponds to the typical structure of peat-gleezem profiles (Fig. 3a). This type of soil is found in depressions between peat mounds of heaving – palza, and cryogenic-ozheleznennye and gley cryozems are developed on the tops of the mounds (the latter are in the soils of medallion spots). Cryogenic heaving is the main process here, complicating the relief and, as a consequence, the soil cover (Fig. 3b). In the lower part of the catena, the soil-forming rocks are the sandy deposits of the slopes and the first fragmentary above-floodplain terrace, and below there is a low floodplain on pebble material. Gley and gray humus soils develop on sandy sediments, alluvial humus soils are common on the pebbles of the low floodplain (Fig. 3a). The depth of seasonal thawing on ancient alluvial sands and pebbles exceeds 2 m, besides, a talik zone is developed near the river's edge, which is why permafrost horizons were not encountered in soil profiles. 3.2. Physico-chemical properties of cryogenic soils of UNP HanoweiThe acidity of water extracts of soils varies in the pH range from 4.7 to 7.5, which corresponds to gradations from acidic to neutral. The surface organogenic horizons of soils differ by more acidic reactions (from 4.7 to 6.2), and by the lower part of the profiles the values increase and reach 7.5. The average pH value is 6.1. Variation within the soil profiles occurs with a coefficient of variation of 12.4% (Table 3). Easily soluble salts are contained in soils ranging from 1.8 to 266 mg/l. The salt content is on average 35.4 mg/l, the quartiles of variation of most concentrations are 8.7 (25%) and 49.1 (75%) mg/l, and significant increases in concentrations (Fig. 4a) are associated with the accumulation of salts in the upper parts of the profiles, where concentrations are 2-5 times higher than the average profile (Table 2). Accordingly, the average values of the electrical conductivity of soils are 70.7 microseconds/cm, the variation occurs in wide ranges from 3.6 to 532 microseconds/cm. The organic carbon content in most of the studied soils is very high due to the fact that organogenic horizons are often represented by peat bogs or tundra litter of a weak degree of decomposition. The maximum contents with org reach 45.8%, and the average content with org in all studied soils is 14.3%. In the upper parts of the profiles, where most of the organic matter is concentrated, and towards the lower part of the profile, the content of organic matter decreases sharply (sometimes by more than 10 times). The variation occurs in the range from 0.5 to 45.8% (Table 3, Fig. 4a). The content of physical clay in the studied soils is on average 33.5%, the lowest content is 6.6% (connected-sandy granulometric composition of the horizon - Table. 1), the highest content is 59.9% (light clay granulometric composition - Table 1). The coefficient of variation of the content of physical clay has a value of about 40% (Table. 3), which indicates a very contrasting distribution of the content of granulometric fractions with diameters less than 10 microns in soil profiles. In the upper part of the catena, soils of sandy loam and loamy granulometric composition are observed (Fig. 3), and in the lower part of the relief profile, soil-forming rocks have a lightweight composition. 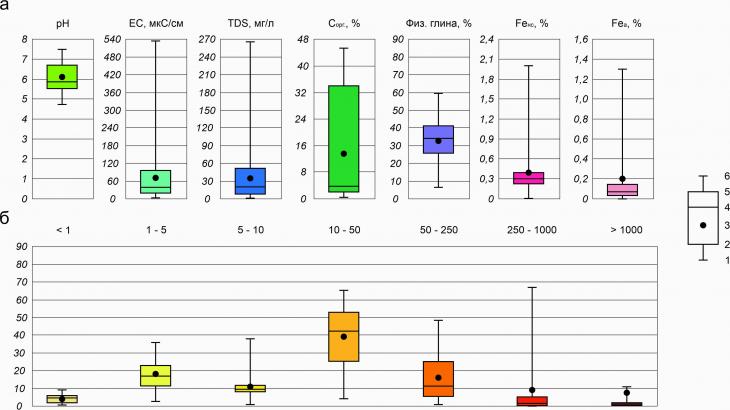 Fig. 4. Physico-chemical properties of cryogenic soils of UNP Hanowei: 1 – minimum value; 2 – quartile 25%; 3 – average value; 4 – median value; 5 – quartile 75%; 6 – maximum value The most common fraction in the studied soils is a fraction with a diameter of 10-50 microns (Fig. 4b), the average content of which is 39.2%, and the distribution itself is closest to normal. Particles with sizes 1-5 and 50-250 microns are also very widespread (average contents are 17.9 and 16.7%, respectively). The largest coefficients of variation are particles with sizes 250-1000 and >1000 microns (186.6 and 346%, respectively) – this fraction is most contrastingly distributed in the soil-geochemical catena, especially particles with sizes >1000, which are generally not found everywhere.
The group composition of iron is mainly represented by a group of silicate compounds that are part of rock-forming minerals. The share of the non-silicate group of iron compounds in the composition of the gross content reaches only 2% (Table. 2), deep mineral horizons of soils are distinguished by such Fe contents, which implies the fractionation of iron in horizons with dynamic indicators of redox potential and active manifestations of the cryogenic cracking process, due to which the crystal lattices of minerals are destroyed and the Fe atoms contained in them become part of other chemical compounds. The proportion of the amorphous fraction, firstly, is very small in the studied soil profiles - up to a maximum of 0.6% (Table. 2), secondly, it is distributed in them almost (0.1–0.3%) evenly (Fig. 4a). Rare exceptions are high values (above 1%) of Fe a, presumably evidence of active interaction of mineral and organogenic fraction of soils at the boundaries of the corresponding horizons. Table 2. Physico-chemical properties of soils of UNP Hanowei Sample No. | Depth, cm | pH | TDS, mg/l | With org., % | Phys. Clay, % | Fe ns, % | Fe a, % | Kh-22-1/1 | 0-3(5) | 5,2 | 81,6 | 35,9 | 14,9 | 0,2 | 0,1 | Kh-22-1/2 |
3(5)-13(34) | 5,0 | 15,7 | 8,5 | 30,4 | 0,4 | 0,1 | Kh-22-1/3 | 13(34)-28 | 6,3 | 5,5 | 1,7 | 33,6 | 0,2 | 0,1 | Kh-22-1/4 | 28-47(80) | 6,2 | 15,4 | 1,6 |
35,2 | 0,3 | 0,0 | Kh-22-1/5 | 47(80)-120 | 7,3 | 22,0 | 4,1 | 28,9 | 0,8 | 0,6 | Kh-22-2/1 | 0-25 | 5,0 | 60,6 | 41,6 | 16,7 | 0,4 | 0,1 |
Kh-22-2/2 | 25-29 | 6,4 | 17,1 | 3,9 | 40,7 | 0,4 | 0,1 | Kh-22-2/3 | 29-43 | 5,2 | 47,5 | 2,0 | 37,5 | 0,3 | 0,1 | Kh-22-2/4 | 43-48 | 5,8 |
58,3 | 0,5 | 32,5 | 2,0 | 1,1 | Kh-22-2/5 | 48-50 | 5,9 | 15,4 | 14,5 | 25,0 | 0,2 | 0,2 | Kh-22-2/6 | 50… | 6,0 | 21,8 | 25,1 | 29,5 | 0,4 |
0,2 | Kh-22-3/1 | 0-1 | 5,5 | 105,0 | 8,5 | 18,1 | 0,3 | 0,1 | Kh-22-3/2 | 1--18 | 7,5 | 52,2 | 13,3 | 29,5 | 0,2 | 0,0 | Kh-22-3/3 | 18-40 |
5,6 | 8,4 | 1,3 | 47,0 | 0,4 | 0,1 | Kh-22-4/1 | 0-2(8) | 5,7 | 33,9 | 9,6 | 30,9 | 0,5 | 0,1 | Kh-22-4/2 | 2(8)-10(19) | 7,1 | 9,8 | 2,3 |
43,4 | 0,5 | 0,3 | Kh-22-4/3 | 10--37 | 6,7 | 6,4 | 1,3 | 53,1 | 0,2 | 0,1 | Kh-22-4/4 | 10(19)-37 | 6,7 | 7,5 | 3,0 | 36,7 | 0,7 | 0,1 |
Kh-22-4/5 | 37+ | 7,2 | 17,7 | 2,7 | 39,2 | 0,5 | 0,0 | Kh-22-5/1 | 0-10 | 6,2 | 63,6 | 45,8 | 35,5 | 0,2 | 0,0 | Kh-22-5/2 | 10--44 | 5,1 |
34,3 | 39,7 | 32,2 | 0,2 | 0,1 | Kh-22-5/3 | 44-66 | 5,6 | 18,2 | 41,3 | 59,9 | 0,3 | 0,1 | Kh-22-5/4 | 66-100 | 5,9 | 9,4 | 35,2 | 40,0 | 0,1 |
0,1 | Kh-22-5/5 | 100-145 | 5,4 | 1,8 | 39,7 | 44,1 | 0,1 | 0,1 | Kh-22-6/1 | 0-1 | 5,7 | 5,5 | 2,0 | 42,0 | 0,2 | 0,1 | Kh-22-6/2 | 1--16 |
5,9 | 6,1 | 1,6 | 45,5 | 0,6 | 0,1 | Kh-22-6/3 | 16… | 6,5 | 5,9 | 0,6 | 26,2 | 0,2 | 0,1 | Kh-22-7/1 | 0-3(7) | 5,8 | 4,0 | 2,4 |
0,0 | 0,3 | 0,1 | Kh-22-7/2 | 3(7)-11(18) | 6,8 | 8,9 | 2,3 | 59,3 | 0,4 | 0,1 | Kh-22-7/3 | 11(18)-70(72) | 6,6 | 5,6 | 2,3 | 57,0 | 0,4 | 0,1 |
Kh-22-7/4 | 70 (72)… | 5,6 | 11,3 | 2,0 | 37,5 | 0,2 | 0,0 | Kh-22-8/1 | 0--2 | 4,7 | 121,0 | 42,9 | 6,6 | 0,1 | 0,0 | Kh-22-8/2 | 2--11 | 5,5 |
50,6 | 35,3 | 16,0 | 1,8 | 0,0 | Kh-22-8/3 | 11-21(23) | 7,3 | 4,5 | 2,1 | 53,2 | 0,6 | 0,1 | Kh-22-8/4 | 21(23)-27 | 6,1 | 23,0 | 5,4 | 36,4 | 0,3 |
0,1 | Kh-22-8/5 | 27-31 | 5,6 | 34,0 | 2,1 | 42,4 | 0,2 | 0,1 | Kh-22-8/6 | 31-45 | 5,3 | 39,0 | 38,4 | 12,0 | 0,4 | 1,3 | Kh-22-8/7 | 45… |
5,0 | 76,3 | 41,9 | 9,0 | 0,1 | 0,0 | Kh-22-9/1 | 0-0,5 | 6,8 | 266,0 | 3,3 | 32,8 | 0,3 | 0,1 | Kh-22-9/2 | 0,5-20 | 6,0 | 59,5 | 32,5 |
12,7 | 0,3 | 0,1 | Kh-22-9/3 | 20… | 6,9 | 23,7 | 3,4 | 34,5 | 0,3 | 0,1 | Kh-22-10/1 | 0--12 | 7,1 | 27,0 | 3,7 | 27,6 | 0,2 | 0,1 |
Kh-22-10/2 | 12… | 7,1 | 20,2 | 8,2 | 16,8 | 2,0 | 1,1 | Table 3. Statistical values of physico-chemical properties of soils of UNP Hanowei Statistical indicators | pH | TDS, mg/l | EU, isS/cm | With org, % | Phys. clay, % | Fe ns, % | Fe a, % | Arithmetic mean | 6,1 | 35,4 | 70,7 |
14,3 | 33,5 | 0,4 | 0,2 | Standard deviation | 0,8 | 45,8 | 91,5 | 16,4 | 13,3 | 0,4 | 0,3 | Coefficient of variation, % | 12,4 | 129,3 | 258,7 | 114,5 | 39,9 | 100,3 |
166,6 | Minimum value | 4,7 | 1,8 | 3,6 | 0,5 | 6,6 | 0,1 | 0,0 | Quartile 25% | 5,5 | 8,7 | 17,3 | 2,1 | 26,9 | 0,2 | 0,1 | Median value | 5,9 |
20,2 | 40,4 | 3,9 | 34,5 | 0,3 | 0,1 | Quartile 75% | 6,7 | 49,1 | 98,1 | 33,9 | 41,4 | 0,4 | 0,1 | Maximum value | 7,5 | 266,0 | 532,0 | 45,8 |
59,9 | 2,0 | 1,3 | Table 4. Granulometric composition of UNP Hanowei soils (percentage of granulometric fractions (microns) in %) | Depth, cm | < 1 | 1--5 | 5--10 | 10--50 | 50--250 | 250--1000 | >1000 | Kh-22-1/1 | 0-3(5) | 1,2 | 7,4 | 6,3 | 39,7 | 41,4 | 4 |
0 | Kh-22-1/2 | 3(5)-13(34) | 3,1 | 15,6 | 11,7 | 62,1 | 7,5 | 0 | 0 | Kh-22-1/3 | 13(34)-28 | 5,6 | 19,4 | 8,6 | 58,5 | 7,9 | 0 | 0 |
Kh-22-1/4 | 28-47(80) | 6,6 | 20,4 | 8,2 | 53,8 | 11 | 0 | 0 | Kh-22-1/5 | 47(80)-120 | 3,2 | 16,8 | 8,9 | 30,7 | 39,3 | 1,1 | 0 | Kh-22-2/1 |
0-25 | 1,1 | 7,6 | 8 | 18 | 26,3 | 38,4 | 0,6 | Kh-22-2/2 | 25-29 | 5,3 | 23,3 | 12,1 | 54,1 | 5,2 | 0 | 0 | Kh-22-2/3 | 29-43 | 5,8 |
21,9 | 9,8 | 57,8 | 4,7 | 0 | 0 | Kh-22-2/4 | 43-48 | 7 | 16,1 | 9,4 | 54,9 | 12,6 | 0 | 0 | Kh-22-2/5 | 48-50 | 2,8 | 13,4 |
8,8 | 50,5 | 24,5 | 0 | 0 | Kh-22-2/6 | 50+ | 2,7 | 15,4 | 11,4 | 57,8 | 12,7 | 0 | 0 | Kh-22-3/1 | 0-1 | 1,7 | 9,5 | 6,9 |
26,8 | 32,7 | 21,9 | 0,5 | Kh-22-3/2 | 1--18 | 2,2 | 14,2 | 13,1 | 18,6 | 9,3 | 39,5 | 3,1 | Kh-22-3/3 | 18-40 | 7,1 | 30,6 | 9,3 | 42,3 |
5,2 | 2,1 | 3,4 | Kh-22-4/1 | 0-2(8) | 4,2 | 17,2 | 9,5 | 46,3 | 22,8 | 0 | 0 | Kh-22-4/2 | 2(8)-10(19) | 6,3 | 26,2 | 10,9 | 53,1 | 3,5 |
0 | 0 | Kh-22-4/3 | 10--37 | 8,7 | 32,3 | 12,1 | 40,8 | 6,1 | 0 | 0 | Kh-22-4/4 | 10(19)-37 | 5,7 | 22,8 | 8,2 | 20,4 | 18,1 | 24,8 |
0 | Kh-22-4/5 | 37+ | 5,2 | 23,2 | 10,8 | 51,3 | 9,5 | 0 | 0 | Kh-22-5/1 | 0-10 | 6,3 | 17 | 12,2 | 28,4 | 32,9 | 3,2 | 0 |
Kh-22-5/2 | 10--44 | 2,4 | 3,5 | 26,3 | 19,5 | 48,3 | 0 | 0 | Kh-22-5/3 | 44-66 | 5,9 | 16,2 | 37,8 | 24,3 | 15,8 | 0 | 0 | Kh-22-5/4 |
66-100 | 5 | 11,2 | 23,8 | 36,4 | 21,7 | 1,9 | 0 | Kh-22-5/5 | 100-145 | 6,5 | 27,1 | 10,5 | 52,9 | 3 | 0 | 0 | Kh-22-6/1 | 0-1 | 6,6 |
25,7 | 9,7 | 53,2 | 4,8 | 0 | 0 | Kh-22-6/2 | 1--16 | 9,1 | 27,9 | 8,5 | 48,9 | 5,6 | 0 | 0 | Kh-22-6/3 | 16+ | 4,3 | 14 |
7,9 | 65,2 | 8,6 | 0 | 0 | Kh-22-7/1 | 0-3(7) | 1,6 | 11,3 | 23,9 | 58,3 | 4,9 | 0 | 0 | Kh-22-7/2 | 3(7)-11(18) | 8,2 | 36,2 | 14,9 |
39,2 | 1,5 | 0 | 0 | Kh-22-7/3 | 11(18)-70(72) | 8,4 | 35,9 | 12,7 | 39,3 | 3,7 | 0 | 0 | Kh-22-7/4 | 70(72)+ | 5,4 | 21,8 | 10,3 | 56 |
6,5 | 0 | 0 | Kh-22-8/1 | 0--2 | 0,5 | 3,1 | 3 | 4,5 | 10,3 | 67,6 | 11 | Kh-22-8/2 | 2--11 | 1 | 7,2 | 7,8 | 16,2 | 28 |
39,6 | 0,2 | Kh-22-8/3 | 11-21(23) | 9,5 | 33,7 | 10 | 43,3 | 3,5 | 0 | 0 | Kh-22-8/4 | 21(23)-27 | 5,2 | 19,7 | 11,5 | 54,8 | 8,8 | 0 |
0 | Kh-22-8/5 | 27-31 | 6,8 | 25,5 | 10,1 | 52,9 | 4,7 | 0 | 0 | Kh-22-8/6 | 31-45 | 0,7 | 5,4 | 5,9 | 16,7 | 36,4 | 34,9 | 0 |
Kh-22-8/7 | 45+ | 0,7 | 4,4 | 3,9 | 5,5 | 14,3 | 60,6 | 10,6 | Kh-22-9/1 | 0-0,5 | 3,7 | 19,3 | 9,8 | 31,7 | 35,4 | 0,1 | 0 | Kh-22-9/2 |
0,5-20 | 0,7 | 5,6 | 6,4 | 49,3 | 38 | 0 | 0 | Kh-22-9/3 | 20+ | 5 | 20,9 | 8,6 | 18 | 40,4 | 7,1 | 0 | Kh-22-10/1 | 0--12 | 3 |
15,6 | 9 | 25,7 | 17,1 | 29,6 | 0 | Kh-22-10/2 | 12+ | 4,8 | 7,2 | 4,8 | 9,7 | 24,6 | 48,9 | 0 | 3.3. Elemental composition of cryogenic soils of UNP HanoweiSilicon and iron, as the main components of the mineralogical basis of the soils of UNP Hanowei, are characterized by the highest average concentrations among all the studied elements. The average Si and Fe contents in soils vary from 2 to 4%, while silicon compounds are distributed more normally in soils (Fig. 5a), and iron compounds are more characterized by very narrow quartiles of distributions and a wider distribution interval in general compared to silicon (Fig. 5a). The remaining macronutrients – Mg, Al, Ca, Ti and Mn – have concentrations not exceeding 1.2%, of which the highest concentrations in Ca (up to 1.18%), Al and Ti are distributed very widely with maximum concentrations reaching 1 and 0.92%, respectively, and average concentrations vary from 0.2 to 0.4%. Mg and Mn have concentrations rarely exceeding 0.1% (Fig. 5a, Table 5). 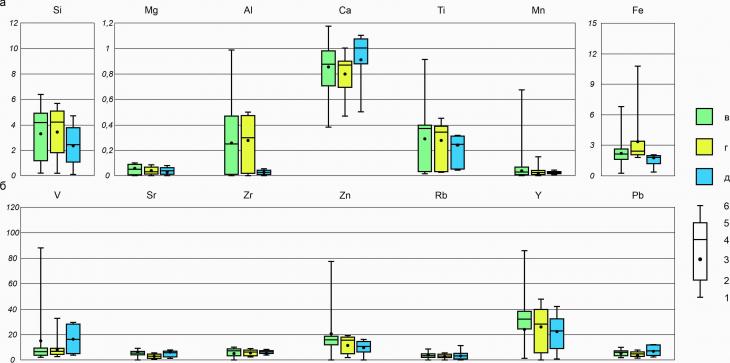
Fig. 5. Gross elemental composition of cryogenic soils of UNP Hanowei: a) macronutrients, b) trace elements; c) seasonally thawed horizons; d) cryogenic seasonally thawed horizons; e) permafrost horizons; 1 – minimum value; 2 – quartile 25%; 3 – average value; 4 – median value; 5 – quartile 75%; 6 – maximum value Among the trace elements, V, Zn and Y are distinguished by the highest concentrations, in the distributions of which the main feature is a very extended range of varying concentrations in the horizons of the seasonally thawed layer without signs of cryogenesis, as well as a wide range of varying concentrations of V and Y in permafrost horizons (Fig. 5b). The remaining trace elements - Sr, Zr, Rb and Pb – are contained in soils in very low concentrations not exceeding 10 mg/kg (Table 6). Among the most pronounced signs of the cryogenic distribution of elements in the soils of UNP Hanowei, there are increases in average (about 1%) Ca concentrations (Fig. 5a), the quartiles of whose contents are also shifted to the upper limits of the content, relative to seasonal thawed and cryogenic seasonal thawed horizons. Among the other macronutrients, the Al content in the MMP horizons differs most significantly from the other two groups of horizons (Fig. 5a). Fe in permafrost horizons, on the contrary, is distributed with decreases in mean values and quartiles of contents to the lower limits. Fe is also distributed in cryogenic seasonally thawed soil horizons with an increase in average concentrations relative to seasonally thawed and permafrost, which is especially noticeable on the example of maximum contents - 11% (compared with seasonally thawed - 7%; and permafrost – 2%). Table 5. Macronutrient content in soils of UNP Hanowei (in %) Cut | Depth, cm | Mg | Al | Si | Ca | Ti | Mn | Fe | Kh-22-1/1 | 0-3(5) | 0,01 | 0,31 | 1,25 | 0,39 | 0,12 |
0,01 | 1,65 | Kh-22-1/2 | 3(5)-13(34) | 0,01 | 0,01 | 4,95 | 0,80 | 0,38 | 0,02 | 1,37 | Kh-22-1/3 | 13(34)-28 | 0,01 | 0,50 | 5,59 | 0,91 | 0,43 | 0,02 |
1,92 | Kh-22-1/4 | 28-47(80) | 0,01 | 0,01 | 5,45 | 1,12 | 0,40 | 0,03 | 2,05 | Kh-22-1/5 | 47(80)-120 | 0,01 | 0,01 | 4,34 | 1,05 | 0,32 | 0,06 | 2,27 |
Kh-22-2/1 | 0-25 | 0,01 | 0,01 | 0,58 | 0,60 | 0,07 | 0,66 | 3,54 | Kh-22-2/2 | 25-29 | 0,01 | 0,37 | 6,24 | 0,82 | 0,45 | 0,03 | 2,57 | Kh-22-2/3 |
29-43 | 0,01 | 0,43 | 4,04 | 0,96 | 0,35 | 0,04 | 3,10 | Kh-22-2/4 | 43-48 | 0,01 | 0,37 | 0,19 | 0,43 | 0,03 | 0,16 | 10,92 | Kh-22-2/5 | 48-50 | 0,01 |
0,01 | 3,69 | 0,98 | 0,36 | 0,06 | 2,44 | Kh-22-2/6 | 50+ | 0,01 | 0,01 | 1,48 | 1,10 | 0,17 | 0,07 | 2,35 | Kh-22-3/1 | 0-1 | 0,01 | 0,49 |
4,21 | 0,70 | 0,34 | 0,04 | 2,92 | Kh-22-3/2 | 1--18 | 0,01 | 0,01 | 2,47 | 0,86 | 0,32 | 0,07 | 2,44 | Kh-22-3/3 | 18-40 | 0,01 | 0,01 | 4,30 |
0,91 | 0,38 | 0,04 | 2,07 | Kh-22-4/1 | 0-2(8) | 0,01 | 0,35 | 4,18 | 0,85 | 0,34 | 0,05 | 2,61 | Kh-22-4/2 | 2(8)-10(19) | 0,01 | 0,42 | 6,03 | 0,86 |
0,41 | 0,04 | 2,70 | Kh-22-4/3 | 10--37 | 0,01 | 0,01 | 3,31 | 0,91 | 0,34 | 0,04 | 2,81 | Kh-22-4/4 | 10(19)-37 | 0,01 | 0,47 | 5,05 | 0,90 | 0,32 |
0,03 | 2,53 | Kh-22-4/5 | 37… | 0,01 | 0,48 | 3,10 | 0,92 | 0,35 | 0,04 | 2,76 | Kh-22-5/1 | 0-10 | 0,01 | 0,23 | 0,90 | 0,99 | 0,32 | 0,02 |
0,80 | Kh-22-5/2 | 10--44 | 0,01 | 0,85 | 1,20 | 0,95 | 0,25 | 0,03 | 0,40 | Kh-22-5/3 | 44-66 | 0,01 | 0,27 | 1,10 | 0,88 | 0,75 | 0,08 | 0,56 |
Kh-22-5/4 | 66-100 | 0,01 | 0,97 | 0,80 | 0,55 | 0,95 | 0,08 | 0,97 | Kh-22-5/5 | 100-145 | 0,01 | 0,13 | 0,40 | 0,74 | 0,48 | 0,05 | 0,83 | Kh-22-6/1 |
0-1 | 0,01 | 0,56 | 4,98 | 0,84 | 0,38 | 0,03 | 2,64 | Kh-22-6/2 | 1--16 | 0,01 | 0,49 | 4,00 | 1,01 | 0,36 | 0,07 | 3,16 | Kh-22-6/3 | 16… | 0,01 |
0,21 | 1,66 | 0,67 | 0,04 | 0,06 | 0,96 | Kh-22-7/1 | 0-3(7) | 0,01 | 0,01 | 4,79 | 0,85 | 0,39 | 0,03 | 2,26 | Kh-22-7/2 | 3(7)-11(18) | 0,01 | 0,01 |
3,09 | 0,80 | 0,35 | 0,03 | 2,68 | Kh-22-7/3 | 11(18)-70(72) | 0,01 | 0,46 | 5,00 | 0,97 | 0,40 | 0,08 | 3,45 | Kh-22-7/4 | 70 (72)… | 0,01 | 0,46 | 5,61 |
0,87 | 0,40 | 0,03 | 2,22 | Kh-22-8/1 | 0--2 | 0,01 | 0,01 | 0,18 | 0,45 | 0,01 | 0,02 | 0,36 | Kh-22-8/2 | 2--11 | 0,01 | 0,25 | 0,20 | 0,44 |
0,01 | 0,15 | 7,04 | Kh-22-8/3 | 11-21(23) | 0,01 | 0,01 | 5,65 | 0,85 | 0,41 | 0,04 | 2,64 | Kh-22-8/4 | 21(23)-27 | 0,01 | 0,50 | 5,17 | 1,11 | 0,36 |
0,06 | 2,20 | Kh-22-8/5 | 27-31 | 0,01 | 0,55 | 4,45 | 0,89 | 0,41 | 0,03 | 2,21 | Kh-22-8/6 | 31-45 | 0,01 | 0,23 | 0,20 | 0,59 | 0,01 | 0,04 |
4,76 | Kh-22-8/7 | 45… | 0,01 | 0,01 | 0,13 | 0,51 | 0,11 | 0,07 | 0,20 | Kh-22-9/1 | 0-0,5 | 0,01 | 0,01 | 4,51 | 1,19 | 0,31 | 0,07 | 2,28 |
Kh-22-9/2 | 0,5-20 | 0,01 | 0,01 | 1,45 | 0,61 | 0,08 | 0,03 | 2,58 | Kh-22-9/3 | 20… | 0,01 | 0,01 | 5,48 | 0,89 | 0,22 | 0,05 | 1,88 | Kh-22-10/1 |
0--12 | 0,01 | 0,01 | 4,60 | 1,09 | 0,38 | 0,02 | 2,39 | Kh-22-10/2 | 12… | 0,01 | 0,01 | 4,35 | 1,02 | 0,30 | 0,05 | 2,26 | Table 6. The content of trace elements in the soils of UNP Hanowei (in g/kg) Cut | Depth, cm |
V | Zn | Rb | Sr | Y | Zr | Pb | Kh-22-1/1 | 0-3(5) | 5 | 0,9 | 1,9 | 4,5 | 0,7 | 6,1 | 3,8 | Kh-22-1/2 | 3(5)-13(34) | 5 | 1,6 | 5,4 |
16,1 | 2,2 | 36,8 | 2,1 | Kh-22-1/3 | 13(34)-28 | 5 | 3,7 | 6,1 | 19,3 | 2,3 | 42,8 | 1,4 | Kh-22-1/4 | 28-47(80) | 5 | 4,9 | 6,1 | 20,4 |
3,4 | 39,8 | 1,7 | Kh-22-1/5 | 47(80)-120 | 29,6 | 4,9 | 5 | 14,3 | 2,1 | 43,4 | 1,7 | Kh-22-2/1 | 0-25 | 5 | 5,5 | 1 | 7,2 | 1,3 |
5,3 | 1,5 | Kh-22-2/2 | 25-29 | 5 | 4,8 | 7 | 17,3 | 2,8 | 39,3 | 1,4 | Kh-22-2/3 | 29-43 | 5 | 5,8 | 6,9 | 18,7 | 3,2 | 33,6 |
5 | Kh-22-2/4 | 43-48 | 5 | 1,3 | 5 | 3,2 | 5 | 1 | 3,1 | Kh-22-2/5 | 48-50 | 26,2 | 7,7 | 5 | 17,3 | 3 | 31,6 | 1,4 |
Kh-22-2/6 | 50+ | 5 | 6,7 | 3,4 | 9,3 | 11,6 | 12,8 | 5 | Kh-22-3/1 | 0-1 | 23,2 | 4,5 | 6,7 | 12,7 | 2,3 | 28,4 | 3,7 | Kh-22-3/2 |
1--18 | 5 | 4,6 | 5,3 | 14 | 2,8 | 27 | 1,1 | Kh-22-3/3 | 18-40 | 5 | 2,8 | 5,7 | 18,3 | 3,2 | 47,9 | 1,4 | Kh-22-4/1 | 0-2(8) | 5 |
4,8 | 5,7 | 16 | 2,4 | 32,2 | 1,6 | Kh-22-4/2 | 2(8)-10(19) | 5 | 4,6 | 6,5 | 17,8 | 3,2 | 35,2 | 5 | Kh-22-4/3 | 10--37 | 27,5 | 4,9 |
7,3 | 18 | 3,1 | 34,6 | 1,8 | Kh-22-4/4 | 10(19)-37 | 5 | 5 | 5,2 | 15 | 2,5 | 24,6 | 5 | Kh-22-4/5 | 37+ | 29,8 | 4,8 | 6,6 |
15,8 | 2,8 | 28,6 | 1,4 | Kh-22-5/1 | 0-10 | 34 | 4,5 | 8,4 | 49,8 | 4,5 | 76,9 | 3,2 | Kh-22-5/2 | 10--44 | 76,5 | 7,8 | 3,4 | 35,1 |
9,6 | 37,5 | 1,6 | Kh-22-5/3 | 44-66 | 3,4 | 2,5 | 7,8 | 78,4 | 2,3 | 28,7 | 6,4 | Kh-22-5/4 | 66-100 | 87 | 9,8 | 2,3 | 52,9 | 7,2 |
75,3 | 5,3 | Kh-22-5/5 | 100-145 | 4,5 | 5,6 | 2,6 | 38,5 | 1,8 | 86,3 | 5,8 | Kh-22-6/1 | 0-1 | 5 | 4,8 | 6,2 | 17,8 | 2,9 | 37,3 |
1,6 | Kh-22-6/2 | 1--16 | 5 | 4,8 | 6,7 | 16,7 | 2,9 | 30,8 | 1,5 | Kh-22-6/3 | 16+ | 5 | 4,8 | 2,3 | 2 | 0,4 | 2,6 | 4,5 |
Kh-22-7/1 | 0-3(7) | 5 | 4,8 | 6,5 | 17,2 | 2,6 | 41,2 | 1,3 | Kh-22-7/2 | 3(7)-11(18) | 5 | 4,8 | 6 | 16,7 | 2,8 | 32,6 | 1,9 | Kh-22-7/3 |
11(18)-70(72) | 5 | 4,8 | 6,8 | 17,5 | 3 | 22,7 | 1,4 | Kh-22-7/4 | 70(72)+ | 34,5 | 4,8 | 6,9 | 18,2 | 2,2 | 41,3 | 1,8 | Kh-22-8/1 | 0--2 | 5 |
4,8 | 0,4 | 1,1 | 1,1 | 1,2 | 8,5 | Kh-22-8/2 | 2--11 | 5 | 4,8 | 5 | 1,6 | 5 | 5 | 4,2 | Kh-22-8/3 | 11-21(23) | 5 | 4,8 |
6,9 | 17,7 | 2,7 | 38,5 | 1,7 | Kh-22-8/4 | 21(23)-27 | 5 | 4,8 | 6,2 | 19,4 | 2,9 | 37,8 | 1,7 | Kh-22-8/5 | 27-31 | 5 | 4,8 | 6,5 |
16,9 | 2,5 | 38,3 | 1,7 | Kh-22-8/6 | 31-45 | 5 | 4,8 | 5 | 15,2 | 2,3 | 27,5 | 5 | Kh-22-8/7 | 45+ | 5 | 4,8 | 5 | 0,9 |
0,9 | 1,1 | 9,6 | Kh-22-9/1 | 0-0,5 | 5 | 4,8 | 5 | 15,2 | 2,3 | 27,5 | 5 | Kh-22-9/2 | 0,5-20 | 5 | 4,8 | 2 | 4,1 | 1,7 |
4,5 | 5 | Kh-22-9/3 | 20+ | 5 | 4,8 | 4,3 | 11,4 | 1,6 | 18,3 | 5 | Kh-22-10/1 | 0--12 | 5 | 4,8 | 4,9 | 14,5 | 2,3 | 40,3 |
1,3 | Kh-22-10/2 | 12+ | 5 | 4,8 | 4 | 11,2 | 1,7 | 33,1 | 1,7 | 3.4. Radial differentiation of cryogenic soils of UNP HanoweiRadial differentiation of concentrations of chemical elements in the soils of UNP Hanowei represents a variety of patterns of profile distributions, among which the following main patterns are distinguished, which are most often encountered: 1) enrichment of most of the cryogenic seasonally thawed soil horizons (Gcf@, CG@, CRg, CRM) with many elements, the concentration of elements with a variable degree of oxidation (Fe, Mn) and some others in gley horizons, or in horizons showing signs of cleavage (G, CRg, O/Cg, Gox and others), as well as relatively uniform distributions of concentrations of most of the elements in the mineral horizons of soils without visually pronounced signs of cryogenesis. The accumulation of macro- and microelements in the surface peat horizons of soils is most likely due to the atmospheric entry of particles containing them to the soil surface as a result of the long-term transfer of industrial emissions of large cities (Vorkuta and its satellite cities) as part of air masses. It is noticeable that peat horizons (T) of higher power have an increased ability to accumulate elements, compared with the horizons of litter (O). While the values of the coefficients R for the litter horizons are most often approximately comparable to the soil-forming rock or even demonstrates scattering (1 ? R) (Fig. 6a, c, d, e, g), approximately two- or more multiple exceedances of Al, Si, Ti concentrations are often observed in peat horizons. Mn, Sr, Y and Zr in the horizons T relative to C (R ? 2). Apparently, the processes of cryogenic structuring in the horizons of CR and CRM soils and the regenerative situation developing in parallel in it during the freezing period (morphological signs of which persist in soils and during the thawing state) contribute to the accumulation of elements such as Mn (R Mn = 2.1) in them (Fig. 6b), Al (R Al = 2,3), Ti (R Ti = 8,9) (Fig. 6e), Rb, Sr, etc. Permafrost horizons of soils rarely create contrasts of profile distributions due to the fact that permafrost horizons (CGcf@, CG@, Gox), showing the functions of radial geochemical barriers with respect to Si, Ti, V, Sr, Zr, etc. metals, contribute to slowing down the vertical migration of substances on the way from the soil surface to the upper boundary of the MMP. 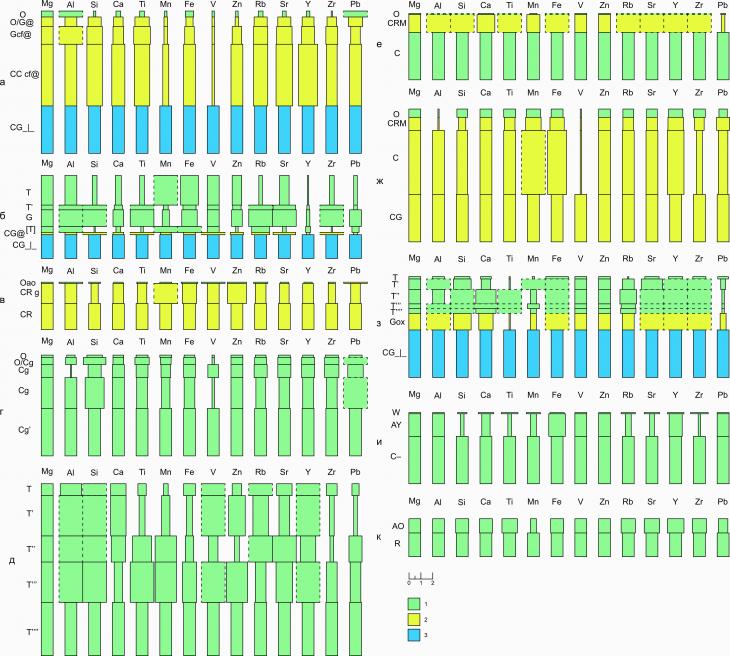 Fig. 6. Radial differentiation of cryogenic soils of UNP Hanowei: a) cryogenic-ozhelezny cryogenic surface- and permafrost-gleevy thixotropic, b) peat-gleezem cryogenic-ozhelezny, c) cryozem gleevy thixotropic, d) cryozem gleevy on ancient alluvial deposits, e) peat oligotrophic soil, e) cryometamorphic gleevy, g) cryometamorphic permafrost-gleevy, h ) peat-gleezem oxidized-gley permafrost, i) alluvial humus soil, k) gray-humus coarse-humus soil on boulder-pebble alluvium; 1 – seasonal-thawed horizons; 2 – cryogenic seasonal-thawed horizons; 3 – permafrost horizons
3.5. Lateral differentiation of cryogenic soils of UNP HanoweiSeasonal thawed organogenic horizons (L Ti = 2.6, L Al = 1.3, L V = 8.2, etc.) of a powerful peat bog (E 2 geochemical landscape) most often act as lateral geochemical barriers to subhorizontal water migration of substances in soil-geochemical catenae on the UNP Hanowei (Fig. 7). Also the concentrations of Mn and Fe in the group of geochemical landscapes of the first above-floodplain terrace are characterized by very contrasting distributions, which is presumably due to the gley environment developed here in peat-gleyozems and cryozems of cryogenic–hardened, gley and gley, and the sharp increase in the contents of Ti, V and Sr (L = 1,7-2,9), it is possible to explain, on the contrary, by aeration of the soil profile of a lighter (sandy) granulometric composition and, accordingly, by the oxidizing medium developed here. Similarly, the compounds of elements mobilized during the reduction in the upper part of the catena are immobilized here when they fall into the sediment as a result of oxidation (Fig. 7). In cryogenic seasonally thawed horizons, lateral differentiation is weakly contrasting in most of the elements. The exception is the increased value of the coefficient L V = 3 in the landscapes of the slope of the second over-floodplain terrace. We associate this manifestation with a change in the lithological composition of soil-forming rocks from peat deposits to cover loams. 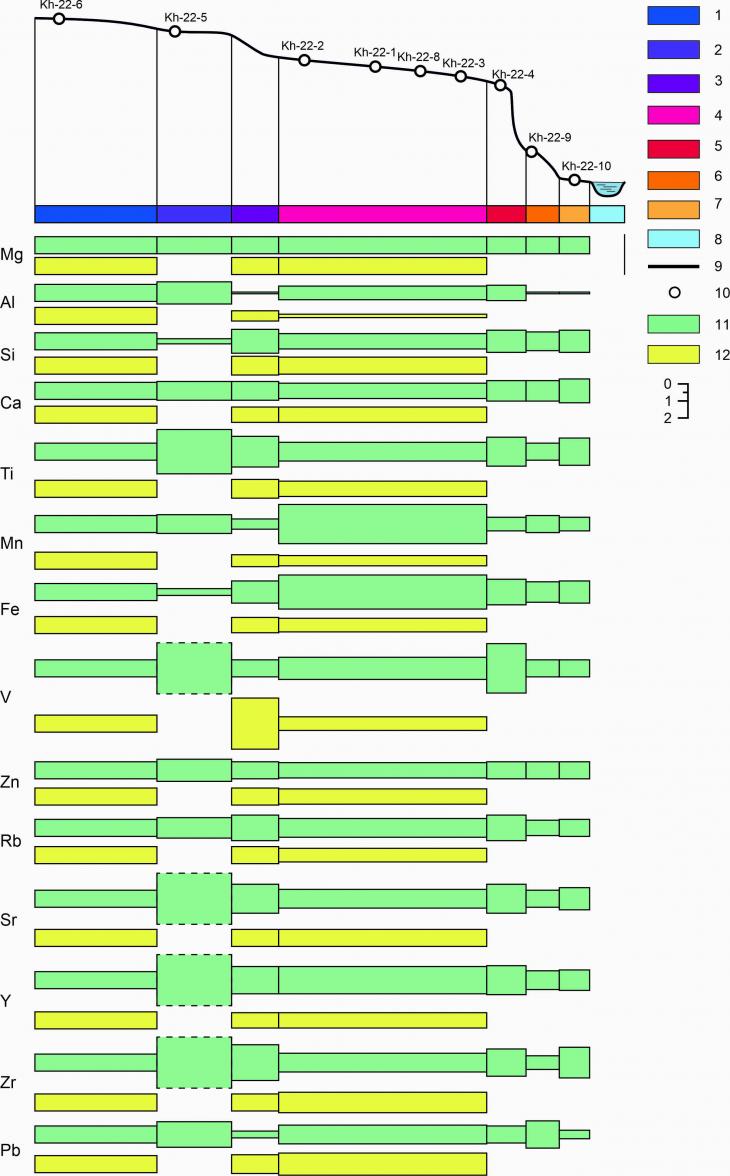 Fig. 7. Lateral differentiation of the chemical composition of cryogenic soils of UNP Hanowei. Elementary geochemical landscapes: 1 – eluvial of the first order (E 1R); 2 – eluvial of the second order (E 2); 3 – transeluvial with weak removal (TE 1); 4 – eluvial of the second order (E2); 5 – transeluvial with active removal (TE 3); 6 – transeluvial-accumulative (TEA); 7 – superqual transit (ST 3); 8 – aquatic (A). Other designations: 9 – relief profile; 10 – locations of soil sections; 11 – seasonal melt horizons; 12 - cryogenic seasonal melt horizons 4. Discussion4.1. Radial distributions The upper horizons of soils have an increased depositing capacity with respect to Fe and Mn (the content of mobile forms of Fe = 176 ± 72.2; Mn = 13.5 ± 8.5, which is 1.5–9 times higher in the horizons A0, A, compared with the horizon Bm) and relatively low – to Ca and Mg (2.5–The content of Bm in horizons is 3 times higher, compared with A0, A), which was observed by D.S. Scriabina in permafrost soils of Yakutia. [21] Similar observations on the concentration of iron and manganese in the organogenic horizons of the soils of UNP Hanowei were made both by us and other researchers in the Bolshezemelskaya tundra.[20] In the south-east of the Bolshezemelskaya tundra, many trace elements differ in removal from the surface organogenic horizons and aeration zones with accumulation at the upper boundary of the gley horizon: Cu (increase from 4-6 to 14 mg/kg), Pb (from 6-8 to 9 mg/kg), Cd (from 0.17 to 0.24 mg/kg), Zn (from 25 to 40 mg/kg), Co (from 4.2 to 10.2 mg/kg), etc.[24] In the soils of the Bolshezemelskaya tundra, the profile distribution of concentrations of trace elements differs in the following features: As – distributed evenly in horizons with high Sorghum contents and oxidizing medium (in the region of 1-2 mg/kg), and it accumulates in the gley horizons, Pb and Cu are distributed relatively evenly in the soil profiles, and Zn is characterized, on the contrary, by biogenic accumulation, which is expressed in the accumulation of this metal in the upper soil horizons [10]. 4.2. Lateral distributionsM.P. Tentyukov[22] determined the following statistical parameters of the distribution of impurity elements (trace elements) in the soils of two main types of landscapes of the southern part of the Bolshezemelskaya tundra – lake-marsh plains and erosive-accumulative plains (Table 7). In automorphic soils of erosive-accumulative plains, the profile distributions of trace elements in soils are quite contrasting with the accumulation of Zr, V and Ti in the middle part of the profile, and Mn accumulates in organogenic horizons. The first phenomenon is most likely associated with the eluvial process, the second with biogenic accumulation. Many elements accumulate at the upper boundary of the thixotropic horizon as a result of precipitation by Fe hydroxides and partial sorption by clay particles. Thus, the combined influence of these two factors on the profile distribution is noted. Table 7. Statistical parameters of the distribution of impurity elements in the landscapes of the southern subzone of the Bolshezemelskaya tundra, g/t (according to [22]) Elements | Lake-marsh plains (multi-lake landscapes), n = 826 | Erosion-accumulative plains, n = 375 |
Q1 | Me | Q3 | KK | Q1 | Me | Q3 | KK | I. Typical cationogenic elements-lithophiles | Sr | 178 | 278 | 464 | 1,0 | 158,5 | 251 | 316 | 0,9 | II. Elements-lithophiles with constant valence |
Y | 10 | 35,9 | 59 | 1,2 | 6,3 | 10 | 15,8 | 0,3 | III. Elements-lithophiles with variable valence | Ti | 3160 | 7700 | 10000 | 2,3 | 630 | 1000 | 3160 | 0,3 | Zr |
100 | 166 | 278 | 1,0 | 39,8 | 63,1 | 15,8 | 0,4 | V | 77,4 | 110,2 | 1,66 | 1,2 | 7,9 | 31,6 | 50,1 | 0,3 | IV. Metals-sulfophiles | Zn |
77,4 | 166 | 215 | 3,9 | 31,6 | 50,1 | 100 | 1,2 | Pb | 10 | 16,7 | 21,5 | 1,4 | 6,3 | 10 | 31,6 | 0,8 | V. Siderophile metals | Mn | 278,3 |
464,2 | 774 | 0,6 | 100 | 199 | 501 | 0,2 | Note: Q1 is the first quartile (25%), Me is the median value, Q3 is the third quartile (75%), KK is the Clark of concentration A slightly different distribution is observed in the profile of surface-gley soils in the transeluvial geochemical landscape of the slope. A weakly expressed thixotropic horizon in the middle part of the soil profile, in which a sign of the cryogenic hardening process is manifested, accumulation of Mn and Zr is noted. In the transaccumulative landscape, the alluvial sod-gley soil is characterized by a relatively weak accumulation of Ti and Zr in the gley horizon G [22, 23]. Tundra soils exhibit the greatest contrast of lateral geochemical barriers with respect to Fe, Mg, Pb, Sr and other metals. Geochemical barriers in subordinate geochemical landscapes are particularly contrasting.[20] 5. ConclusionCryogenic soils were studied at the Khanovey educational and scientific training ground, located 30 km south of Vorkuta in the Komi Republic. The physico-geographically studied area belongs to the southwestern part of the Bolshezemelskaya tundra and the soils of this area were classified as cryozems, peat-gleezems, cryometamorphic, alluvial and gray humus. They are more likely to show signs of cryogenic ozheleznenie, oglenie, etc. processes. Soils are acidic and close to neutral in acidity reactions, the content of water-soluble salts in them most often does not exceed 100 mg / l, the content of Sorghum reaches 45%, since peat accumulation is extremely active here. According to the granulometric composition, the soil horizons vary from sandy to heavy loamy and light loamy, a sharp change in the granulometric composition of the soil-forming rocks occurs during the transition from the surface of the first floodplain terrace of the Vorkuta River to its slope. The gross composition of iron is mainly represented by a group of silicate compounds, the non-silicate fraction reaches concentrations in the region of 2%, and the amorphous fraction is generally small. Among the patterns of radial differentiation of concentrations of chemical elements in the soils of UNP Hanowei , the following are distinguished: 1) most of the cryogenic seasonally thawed soil horizons are enriched with many elements, 2) the concentration of elements with a variable degree of oxidation in the gley horizons, 3) relatively uniform distribution of concentrations in the mineral horizons of soils without signs of cryogenesis. Peat horizons (T) of higher power have an increased ability to accumulate elements, compared with litter horizons (O). R for litter horizons are most often comparable to the soil-forming rock or even demonstrates scattering. The processes of cryogenic structuring in the horizons of CR and CRM soils and the regenerative environment developing in parallel in it contribute to the accumulation of Mn (R Mn = 2.1), Al (R Al = 2.3), Ti (R Ti = 8.9), Rb, Sr, etc. in them. Seasonal thawed organogenic horizons (L Ti = 2.6, L Al = 1.3, L V = 8.2, etc.) of a powerful peat bog (E 2 geochemical landscape) act as lateral geochemical barriers in soil-geochemical catenae. Mn and Fe are also characterized by very contrasting distributions in the landscapes of the first floodplain terrace, and a sharp increase in the contents of Ti, V and Sr (L = 1,7–2,9) in them, on the contrary, is associated with aeration of the soil profile of the sandy granulometric composition. In cryogenic seasonally thawed horizons, lateral differentiation is weakly contrasting in most of the elements. The soil-geochemical structure of the southern tundra landscapes of the south-east of the Bolshezemelskaya tundra in the valley of the Vorkuta River has been studied in detail. These data make it possible to clarify the features of the functioning of geochemical landscapes of a number of regions of the Russian Arctic and Subarctic.
References
1. Atlas of Soils of the Komi Republic, Ed. G.V. Dobrovolsky, A.I. Taskaeva, I.V. Downhole. (2010). Syktyvkar: Komi Republican Printing House LLC, 356 p. (in Russian).
2. Bogdanova M.D., Gavrilova I.P., Gerasimova M.I. (2012). Elementary landscapes as objects of landscape geochemical mapping. Vestn. Moscow university Ser. 5. Geography, No. 1, 23-28. (in Russian).
3. Budantseva N.A., Gorshkov E.I., Isaev V.S., Semenkov I.N., Usov A.N., Chizhova Ju.N., Vasil'chuk Yu.K. (2015). Engineering geological and geochemistry features of the palsa and lithalsa landscape in the area of educational and scientific polygon Khanovey. Engineering Geology. N3. P. 34–50. (in Russian).
4. Vasil'chuk Yu.K. (2013) Modern southern limit of permafrost in Western Siberia lowland. Earth's Cryosphere (Kriosfera Zemli), 1917), 17–27. (in Russian).
5. Vasil'chuk Yu.K., Ginzburg A.P., Budantseva N.A., Vasil'chuk J.Yu. (2022). Cryogenic soils on the Khanovey educational field training site, Vorkuta district, Komi Republic. Arctic and Antarctic, 3, 92–128. doi: 10.7256/2453-8922.2022.3.39001. (in Russian).
6. Geobotanical zoning of the Non-Chernozem region of the European part of the RSFSR. (1989). Leningrad: Nauka, 64 p.(in Russian).
7. Geocryology of the USSR. European territory of the USSR (1988). Ed. E.D. Ershov. Moscow: Nedra, 357 p. (in Russian).
8. Geological and geomorphological maps (1964). / B.I. Guslitser, V.A. Raznitsyn, M.V. Fishman, G.A. Chernov. Atlas of the Komi ASSR.-Moscow: 9-18. (in Russian).
9. Devyatova E.I. (1963). On the marginal formations of the Valdai Glacier on the territory of the Arkhangelsk Region. In Proceedings of the commission for the study of the Quaternary period, vol. 21.-Moscow: Publishing House of the Academy of Sciences of the USSR, 1963, 5-15. (in Russian).
10. Dymov A.A., Lapteva E.M., Kalashnikov A.V., Deneva S.V. (2010). Background content of heavy metals, arsenic and hydrocarbons in the soils of the Bolshezemelskaya tundra. Theoretical and applied ecology,4,43-48. (in Russian).
11. Classification and diagnostics of Russian soils (2004). Authors and compilers: L.L. Shishov, V.D. Tonkonogov, I.I. Lebedeva, M.I. Gerasimov.-Smolensk: Oikumena, 342 p.(in Russian).
12. Climate of Vorkuta: http://www.pogodaiklimat.ru/climate/23226.htm (Accessed 03/21/2023). (in Russian).
13. Forests of the Komi Republic. (1999). / G.M. Kobuzov, A.I. Taskaev, S.V. Degteva and others. Moscow: Design. Information. Cartography, 332 p. (in Russian).
14. Osadchaya G.G. (2017). Features of landscape indication of geocryological conditions in the Bolshezemelskaya tundra. In Proceedings of the XII International Landscape Conference "Landscape Science: Theory, Methods, Landscape and Ecological Support of Nature Management and Sustainable Development" (Tyumen-Tobolsk, August 22-25, 2017).-Publishing House of the Tyumen State University, V. 2, 389-391. (in Russian).
15. Perelman A.I. (1975). Geochemistry of the landscape. Moscow: Higher. school, 1975. 394 p. (in Russian).
16. Perelman A.I., Kasimov N.S. (1999). Geochemistry of the landscape. Moscow: Astreya, 1999. 764 p. (in Russian).
17. Field determinant of soils. (2008). Moscow: Soil in-t im. V.V. Dokuchaeva, 182 p. (in Russian).
18. Vegetation of the European part of the USSR (1980). Ed. S.A. Gribova, T.I. Isachenko, E.M. Lavrenko.-Leningrad. Nauka, 429 p.
19. Semenkov I.N. (2016). Forms of finding metals in loamy tundra, taiga, subtaiga and forest-steppe soil-geochemical catenas / Abstract of the dissertation for the degree of Candidate of Geographical Sciences. Moscow: 24 p. (in Russian).
20. Semenkov I.N., Kasimov N.S., Terskaya E.V. (2016). Lateral distribution of metal forms in tundra, taiga, and forest-steppe catenas of the East European Plain, Vestn. Moscow University. Ser. 5. Geography,3, 29-39.(in Russian).
21. Scriabina D.S. (2016). The state of iron compounds in permafrost-taiga soils / Dissertation for the degree of candidate of biological sciences. Federal State Budgetary Institution of Higher Education RGAU-Moscow Agricultural Academy named after K.A. Timiryazev. Moscow: 224 p. (in Russian).
22. Tentyukov M.P. (2010). Geochemistry of plain tundra landscapes (on the example of Yamal and Bolshezemelskaya tundra).-Syktyvkar, 260 p. (in Russian).
23. Tentyukov M.P. (2013). Features of the distribution of chemical elements in frozen soils. Earth's Cryosphere, 3(XVII), 100-107.(in Russian).
24. Shakhtarova O.V. (2016). Trace elements in the structural components of the soils of the southeast of the Bolshezemelskaya tundra. In Proceedings of the XXIII All-Russian Youth Scientific Conference (with elements of a scientific school) "Actual problems of ecology and biology" (Syktyvkar, April 04-08, 2016). Institute of Biology, Komi Scientific Center, Ural Branch of the Russian Academy of Sciences, 2, 133–138. (in Russian).
25. Oberman N.G., Mazhitova G.G. (2001). Permafrost dynamics in the Northeast European Russia based on the period pf climatic warming 1970–1975. Norsk Geografosk Tidsskrift – Norwegian Journal of Geography, 55(4), 241–244. doi: 10.1080/0029195015274659.
Peer Review
Peer reviewers' evaluations remain confidential and are not disclosed to the public. Only external reviews, authorized for publication by the article's author(s), are made public. Typically, these final reviews are conducted after the manuscript's revision. Adhering to our double-blind review policy, the reviewer's identity is kept confidential.
The list of publisher reviewers can be found here.
The subject of the study, according to the author, is the geochemical composition of cryogenic soils and its spatial differentiation on the example of the Khanovey educational and scientific landfill in the Bolshezemelskaya tundra. The methodology of the study, the article indicates the characteristics of the landfill, based on the analysis of the article, it can be concluded that the methods of sample analysis are used in the chemical analytical laboratory of the Ecological and Geochemical Center (EGC) of the Faculty of Geography of Moscow State University (Moscow) analytical indicators of soils: acidity of aqueous extract (pH), total content of easily soluble salts (TDS), total the content of organic carbon (Sorg.), the granulometric composition of soils, the concentrations of non-silicate (Feh) and oxalate-soluble (Fea) iron in soils, as well as the gross content of macro- and microelements., as well as the analysis of literary data. The relevance of the topic raised is unconditional and consists in obtaining information about the melting of permafrost rocks as a result of climatic changes is especially pronounced in the area of the southern boundary of the cryolithozone, an increase in the capacity of the seasonally thawed soil layer from year to year contributes to an increase in the depth of active migration of chemicals and a decrease in the contrast of profile distributions of the content of chemical elements. This allows you to identify the consequences for changing weather and climatic conditions. The scientific novelty lies in the attempt of the author of the article, based on the conducted research, to conclude about a comprehensive study of permafrost rocks. This is an important direction in the development of geocryology. Style, structure, content the style of presentation of the results is quite scientific. The article is provided with rich illustrative material reflecting the physico-chemical properties of cryogenic soils of different fractions. The author classified the soils of this area as cryozems, peat-gleesems, cryometamorphic, alluvial and gray humus, considered in detail the elemental composition of cryogenic soils, radial and lateral differentiation of the chemical composition of cryogenic soils, determined the statistical parameters of the distribution of trace element impurities, which makes the results presented by the author of the article very interesting. However, there are a number of issues, in particular: The author of the article should highlight the sections of the article for a better perception of the purpose and objectives of the study. The author should check stylistic and spelling inaccuracies (for example: "These data make it possible to clarify the features of functioning ..."). Of the directions given by the author, it should be noted that it is particularly interesting, in addition to studying the features of the functioning of geochemical landscapes in a number of regions of the Russian Arctic and Subarctic, attention should be paid to the rate of migration and the volume of geochemical processes, the processes of soil change, the background of changes in climatic conditions. The bibliography is very comprehensive for the formulation of the issue under consideration, but does not contain references to methodological recommendations and technological features of soil and soil analysis. The appeal to the opponents is presented in identifying the problem at the level of available information obtained by the author as a result of the analysis. Conclusions, the interest of the readership in the conclusions there are generalizations that made it possible to apply the results obtained. The target group of information consumers is not specified in the article.
|